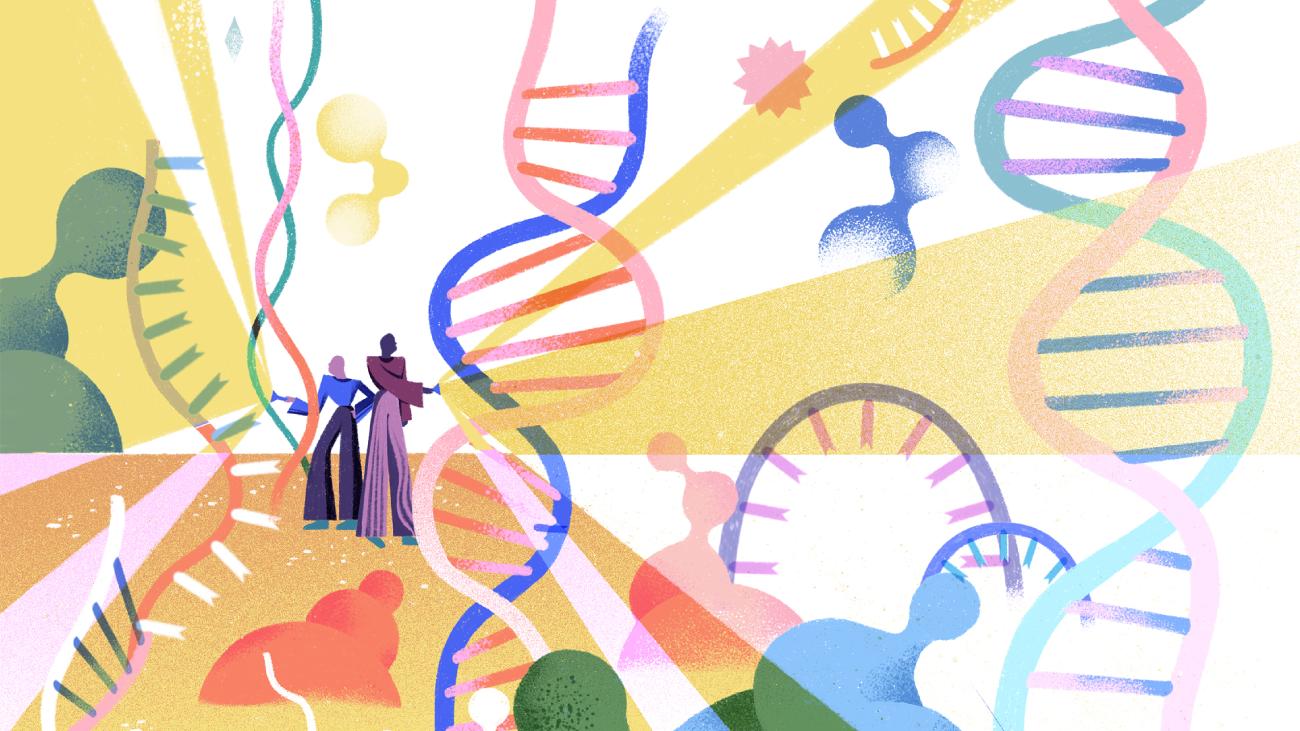
Illustrations by Marina Muun.
The long and winding code
How CRISPR gene-editing technology is changing the future of health sciences.
“The future is here,” the saying goes, “it’s just not evenly distributed yet.”
There’s a good chance the last sci-fi movie you watched included an element of the fantastical that is already within our reach. To use the Star Trek yardstick, we may not have transporters and warp drive, but androids are old news, and if you own a Fitbit, you’re basically wearing a tricorder.
In the field of medical science, technology we associate with future generations is often already in play. Breaking scientific barriers is only half the battle – the real challenge is scaling technology for entire populations. One of the largest gulfs between a technology that’s currently available and the ability for the general public to access it can be found in CRISPR applications, which use a revolutionary gene-editing technique that allows us to alter the very code of our existence.
CRISPR technology (technically called CRISPR-Cas9) is based on a natural virus-defense system used by some bacteria, in which a protein slices into a genome and alters the genetic code of a living organism with tremendous precision. “Bacteria can be infected with viruses, so they developed a particular machinery that basically cuts the viruses into little pieces,” explains Josef Penninger, director of UBC’s Life Sciences Institute and the Canada 150 Chair of Functional Genetics.
“This is basically the bacterial immune system, and we use this bacterial machinery to cut into genes, to rapidly engineer genomes, to inactivate genes, to make single-point mutations in genes.”
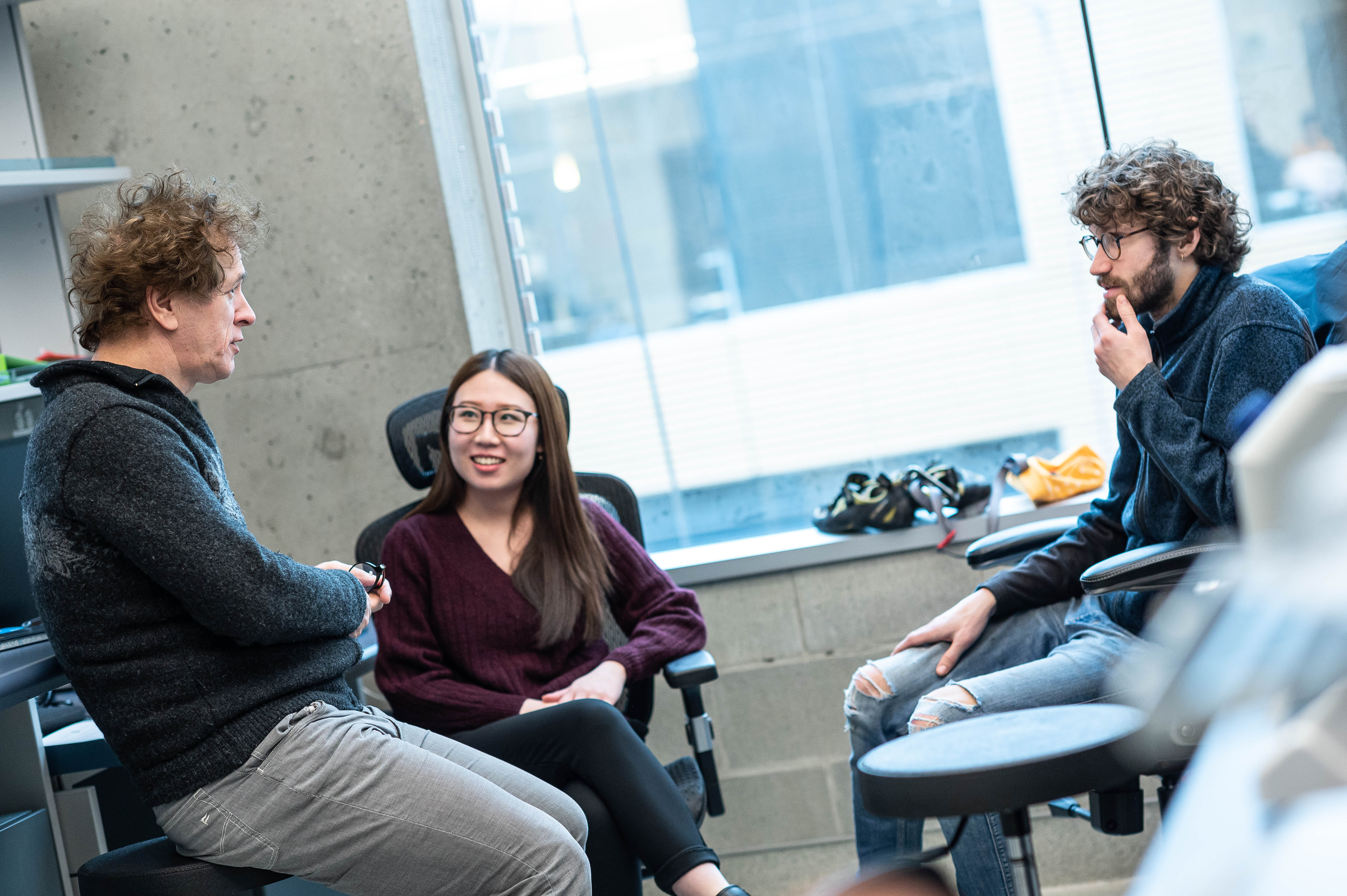
The DNA sequences that comprise the CRISPR family are known as Clustered Regularly Interspaced Short Palindromic Repeats (CRISPR), and in less time than it takes to decipher that term, a CRISPR platform can combine those sequences with a Cas9 protein to cut into a cell’s genome and add or remove genes. “And it’s not just engineering the genome in human cells or mouse cells,” adds Penninger. “It can be done essentially to every organism on the planet.”
The scope is almost beyond belief. CRISPR technology has already been used to engineer probiotic cultures in yogurt, to modify yeast for biofuels, to cure malaria in mosquitos, and to create hardier, more nutritious farm crops.
The biomedical applications of gene editing are limited only by the imagination. Any malady that has a genetic component – either by birth or by mutation – has the potential to benefit from CRISPR gene-editing techniques. While many illnesses have environmental as well as genetic factors, being able to control the genetic contributor to Huntington’s disease, cystic fibrosis, muscular dystrophy, cancer and heart disease, just to name a few, can change the face of public health.
Penninger wants to see that change happen at UBC, by building a CRISPR super-platform that will help medical researchers expedite the development of treatments for gene-related medical conditions, and work at the forefront of the rapidly evolving technology.
It would be a major advancement in a scientific field that can barely keep up with the string of major advances it’s lately enjoyed. Genomic science is barely out of diapers compared to other scientific fields. It’s only 150 years since Gregor Mendel founded the field of genetics, less than 70 since we identified the structure of DNA, and 25 since the first cloned mammal. If you remember Dolly the sheep, you are older than the modern age of genetics.
But these were passive applications – reading a book is one thing, writing a book is something else entirely. “It's really the first time in our history where we don’t have to just sit by and observe nature, to set up the experiment to see what happens,” says Penninger. “We can actively change the genetic setup of organisms, from flies to worms to mosquitoes to, of course, humans – all with the promise of new human medicines.”
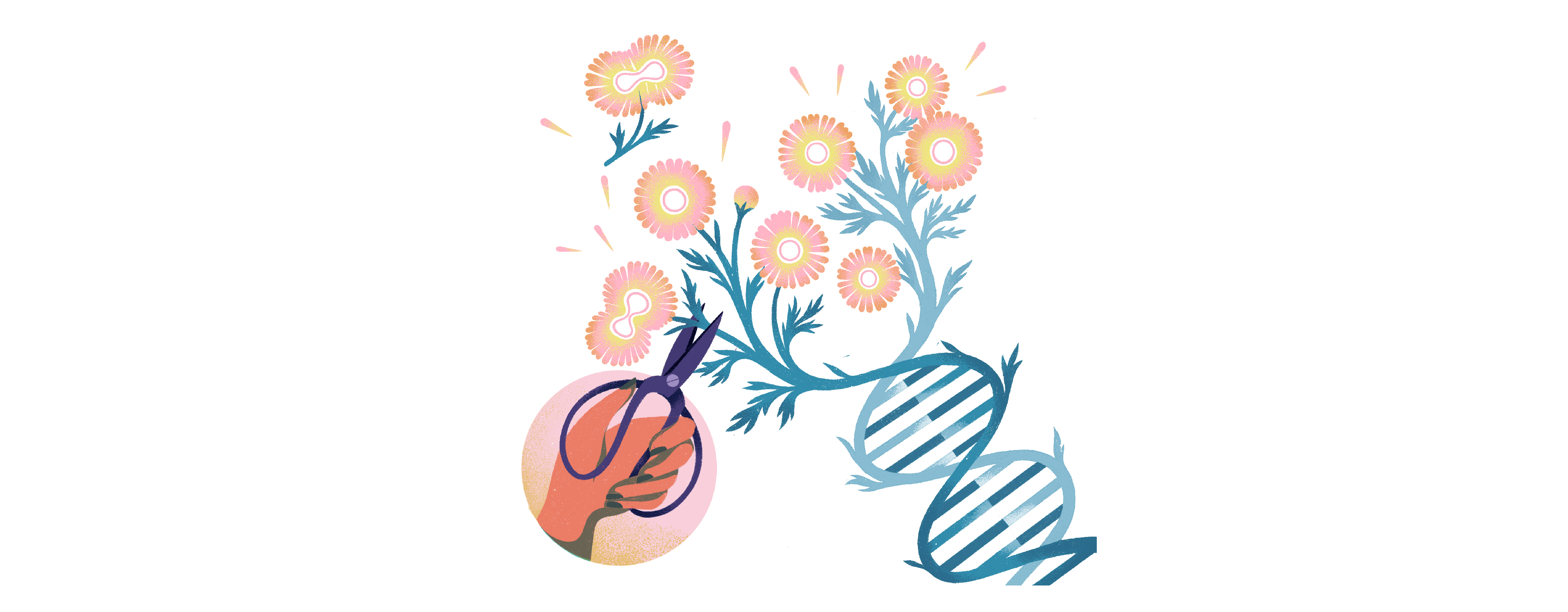
In terms of impact on healthcare, medicines are only the beginning. CRISPR tech has been able to turn adults’ cells into stem cells, essentially turning back time. Researchers can then use those stem cells to create new tissues, which could eventually lead to the ability to grow entirely new organs genetically tailored for the individual. At the Institute of Molecular Biotechnology of the Austrian Academy of Sciences in Vienna, which Penninger led as the founding scientific director from 2002-2018, researchers are already growing blood vessels, heart components, and “mini-brains” that help researchers observe and understand how cancers develop.
But what matters is where the technology has its true impact – in patient care. The very concept of “precision medicine” is rare in healthcare, with the greatest resources generally steered towards the broadest applications. Gene-editing technology opens the door to treatments for a host of rare diseases that might be cured in an afternoon.
“I think the first applications clearly will be in this rare, single-patient scenario,” says Penninger. “Because the common diseases – diabetes, Alzheimer's, Parkinson's – most of these diseases are multifactorial. It would be nearly impossible just to change one gene and we won't have Alzheimer's anymore or diabetes anymore. So the first people benefiting from these new technologies will be people with rare deadly diseases.”
Penninger tells the story of two siblings with a rare anemia that prevents them from making enough red blood cells, which the body needs to transport oxygen to the organs. Because this is caused by a genetic mutation, his team was able to use CRISPR techniques in stem cells to repair the underlying gene mutation and then create new red blood cells to model the disease process and test which genes are responsible.
The father of the children has the same mutation, but he is perfectly healthy. So they took cells from the father, brought them back into a stem-cell-like stage, and compared them side-by-side with his children’s stem cells. Experiments like this help them to understand the disease on an entirely new level, potentially leading to a solution as simple as turning off a light switch.
But who pulls those switches? How do we know when we are crossing a line when that line is microscopic, and the options infinite?
Penninger quotes Dostoevsky's The Brothers Karamazov, where he called psychology “a stick with two ends...it all depends on whose hands it is in.” That’s where we are with genetic science today, and since the genie is out of the bottle, that’s a place we’re going to have to get comfortable with.
“I think we all have to be aware we are tampering with technology, with nature, and there will be some accidents. But in the grand scale I think this is clearly technology that will advance biomedical research and medicine and advance our life on this planet.”
It’s a question we’ve struggled with since the dawn of technology – what risks are worth what rewards?
Until just a few hundred years ago, this was a local question, affecting only those within the grasp of the decision-maker’s reach. Since the industrial revolution, into the nuclear age, and now with the ability to literally program our environment, it’s become a global one, where the future of the human race – for better or worse – could hinge on a single strand of modified DNA.
CRISPR technology has already been used to engineer probiotic cultures in yogurt, to modify yeast for biofuels, to cure malaria in mosquitos, and to create hardier, more nutritious farm crops. The biomedical applications of gene editing are limited only by the imagination.
How do we maximize the medical benefits of this biotech while preventing its misuse? How do we navigate the impossibly complicated regulations (and lack thereof) across 200-plus nations that can’t even agree to stop making disposable plastic? How do we avoid starring roles in Jurassic Planet?
“Much of the challenge that we face in terms of policy, including CRISPR, is that whatever we find out about the magnitude and probability of risks and benefits, the choice about what counts as important and how they weigh against each other is not a scientific choice,” says Michael Burgess, a biomedical ethicist at UBC’s School of Population and Public Health who has been studying genetics for more than 25 years.
“Ethical theory doesn't determine that either. These are effectively political choices, and we have to involve an informed public in the discussion.”
And just how far is an informed public willing to take it? Almost universally, Burgess says, people are more risk tolerant for technologies that address human health than they are for advances in environment and food systems. Splitting genes to solve diseases sounds a lot sexier to people than monkeying around with the food supply.
So when it comes to our health, not to mention our future genetic lines, how far down that slippery slope are we willing to go before digging in the spikes?
It’s a tough question to answer if the slope keeps changing shape. “Science moves incrementally in terms of its accumulation of knowledge,” says Burgess, “but it also tends to move social policy incrementally.”
It wasn’t long ago that in-vitro fertilization (IVF) procedures were banned or unavailable in some countries, and, even if available, weren't covered by insurance. But as society more fully embraced having children as a natural right, IVF became a common feature in healthcare coverage.
CRISPR applications are likely to be heading down the same slope, from a novelty few can access to a human right under the principle of equitable healthcare for all. Inevitably that means we will be deliberately changing the very nature of our descendants.
“Whenever we're assessing technology, there's the intended consequences, the thing we're designing something to do,” says Burgess. “And gene editing is an improvement over other forms of genetic modification, because it's much more precise in replacing a sequence in a certain place. What we don't know is what the effect of that is on the entire organism and over generations. So the question is, how far do we go? And then what do we do when we release that from a laboratory into a situation where the environment acts upon it?”
For the most part, we tend to accept that nature itself is unjust. It doesn't fairly distribute benefits and burdens, and over the course of history we have always intervened to figure out how we can better distribute our resources, the benefits of healthcare, and the risks of the technology we implement. But we are imperfect at assessing our own fallibilities, so often society has to make some guesses and take some chances. And usually the consequences of those guesses and chances are passed down to future generations.
Often – as we have been recently reminded – this is for the better.
No other organization in Canada contributed to solving the COVID pandemic as much as UBC and the Life Sciences Institute, largely the benefit of work done years before. Pieter Cullis, who served as LSI’s director before Penninger, developed the nanoparticles used in the Pfizer and Moderna vaccines more than a decade ago. UBC adjunct professor Carl Hansen and colleagues co-founded AbCellera – Canada’s most valuable biotechnology company – and developed the first antibody treatment for the disease based on work done at the university’s Michael Smith Laboratories in 2012. And Penninger’s own work almost 20 years ago, before he arrived at UBC, established that the ACE2 enzyme found on the surface of most cell walls is the gateway through which viruses – including SARS-CoV – infect humans. Today, he is leading investigations into some promising anti-viral drug therapies targeting SARS-CoV-2, which causes COVID-19.
“Developing the vaccines we've got right now was a spectacular triumph of science,” says Penninger. “It’s quite remarkable. And there would have been no way without Pieter Cullis’ work that vaccines would have been developed that fast. You never know what, in 10 or 20 years, might save our planet.”
This is why Penninger thinks the time is ripe to develop in North America the type of organization he grew at the Institute of Molecular Biotechnology in Vienna, considered one of the premier research facilities in the world. By transplanting the model to UBC, he envisions building a CRISPR hub available to researchers from any field of science, offering a skeleton key to crack any genetic puzzle on earth.
“A super-platform will help any researcher working on any organism to get help from a team of expert technicians who are permanently up to date with the latest technologies,” he says. “At the end of the day, CRISPR is CRISPR. You can adjust the basic technology to flies or humans to wheat. This really will allow everybody from every field to be faster and better.”
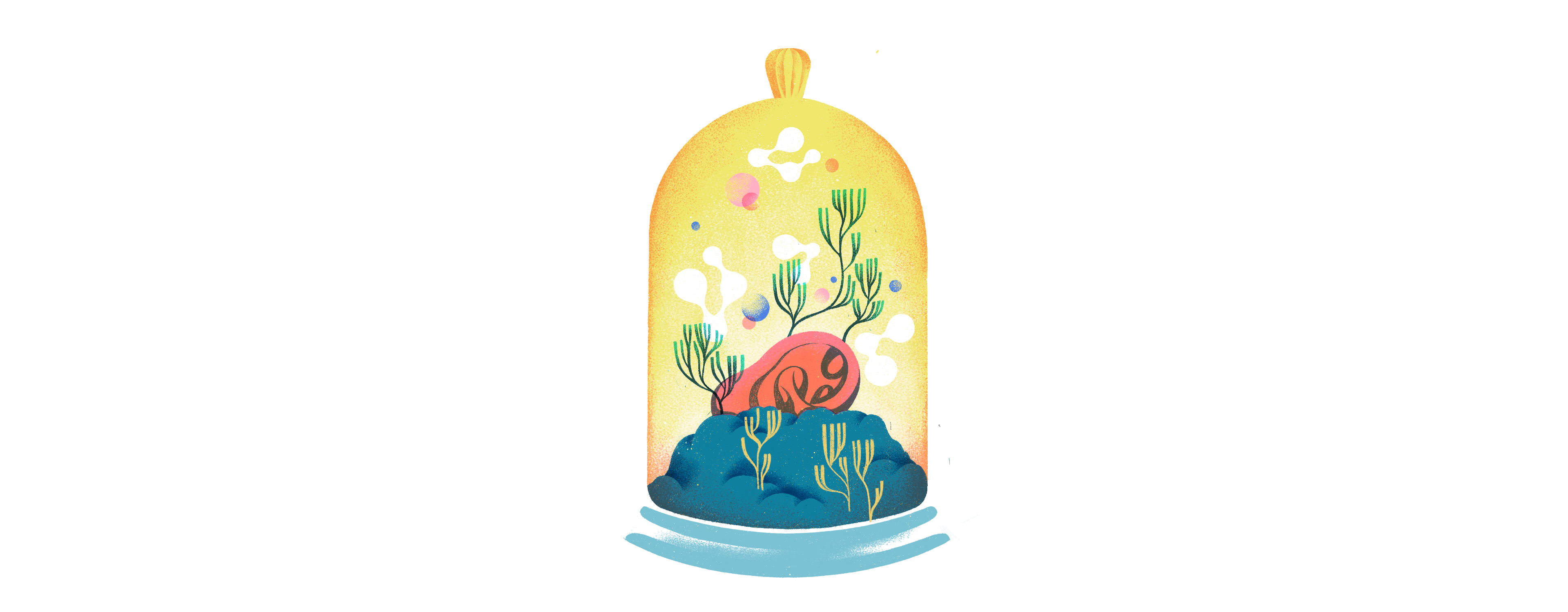